QUANTUM EFFECTS OF POLAR MOLECULES AT ABSOLUTE ZERO
A novel technique to chill gases containing polar molecules to close to absolute zero has been discovered by researchers at the Max Planck Institute for Quantum Optics. This opens up the possibility of researching the quantum effects of unusual types of materials.
At MPQ, researchers have created a brand-new method of cooling molecular vapours. Polar molecules can be cooled to a few nanokelvin thanks to it.
The researchers team used a spinning microwave field as their secret weapon to get past this obstacle. Through the use of an energy shield, it aids in stabilising the molecular collisions that occur during cooling. The Max Planck scientists were able to chill a gas of sodium-potassium molecules to a temperature that was 21 billionths of a degree above absolute zero in this manner. They did this, breaking the previous low-temperature record. Future research will be able to build and examine a variety of quantum matter types that have not yet been made experimentally accessible.
A highly diluted gas exhibits strange features when chilled to incredibly low temperatures. As a result, some gases can create a sort of matter known as a Bose-Einstein condensate, in which all atoms move in unison. Another illustration is supersolidity, which is a state of matter where it acts like a fluid with no friction and a regular structure. When chilling gases made up of polar molecules, physicists anticipate discovering exceptionally varied and illuminating types of quantum matter. They are distinguished by an unbalanced distribution of electrical charge. They may rotate, vibrate, and either attract or repel one another, unlike unbound atoms. Molecular gases, however, are challenging to cool to extremely low temperatures. Now, a group of scientists from the Max Planck Institute of Quantum Optics (MPQ) together with other researchers in the field of quantum optics has discovered a quick and efficient solution to this challenge. It is based on a field of microwaves that rotates.

Laser-induced confinement of sodium-potassium (NaK) molecules in an optical trap
The scientists employed a gas of sodium-potassium (NaK) molecules that were contained in an optical trap by laser light for their studies. The scientists used a technique known as "evaporative cooling," which has a long history of success in cooling unbound atoms, to cool the gas. According to researchers in the field of quantum optics, the Laboratory for Ultracold Polar Molecules in the Division of Quantum Many-Body Systems : This technology works similarly to the familiar procedure, which allows a cup of hot coffee to cool down. Water molecules regularly collide in coffee, exchanging some of their kinetic energy in the process. When two molecules with high energy collide, one of them can accelerate to the point that it can steam out of the coffee. With less energy, the other molecule is still present. The coffee progressively cools down in this manner. Similar to this, at minus 273.15 degrees Celsius, a gas can be chilled to a few nanokelvin, or billionths of a degree above absolute zero.

However, according to researchers in this field - If the gas contains molecules, these must be further stabilised at very low temperatures. The difference in complexity between the structure of molecules and that of free atoms explains this. It is therefore challenging to control their motions when they collide. When the molecules collide, they may stick together. Researchers in the field of quantum optics noticed that polar molecules behave like small magnets that can snap together, in which case they are lost for the experiment. These challenges have emerged as a major barrier to study in recent years.
Electromagnetic field that protects molecules from energy by acting as a shield
The researchers in the field of quantum optics used a cunning tactic to get around this problem: they applied an additional electromagnetic field that was carefully created to act as an energy shield for the molecules, preventing them from adhering to one another. Andreas Schindewolf explains, "We used a strong rotating microwave field to build this energy shield. The molecules rotate with a higher frequency as a result of the field. If two molecules approach one another too closely, they can exchange kinetic energy as a result, but they also align in such a way that they repel one another and swiftly separate once more.
The scientists positioned a helical antenna beneath the optical trap holding the gas of sodium-potassium molecules in order to generate a microwave field with the necessary characteristics. According to Xin-Yu Luo, "the rate at which the molecules were entangled was therefore reduced by more than one order of magnitude." A potent and extensive electrical interaction between the molecules also emerged while the field was in play. The physicist claims that as a result, they clashed significantly more frequently than they would have otherwise-on average, 500 times each molecule. That was sufficient to evaporatively cool the gas to nearly absolute zero.
Nearly 21 nanokelvin, or considerably below the crucial "Fermi temperature"
After just a third of a second, the temperature reached around 21 nanokelvin - well below the critical "Fermi temperature". It marks the limit, below which quantum effects dominate the behaviour of a gas - and bizarre phenomena start to arise. The temperature that has been reached is the lowest so far in a gas of polar molecules - according to the researchers in the field of quantum optics. And the researchers opine that they can reach even far lower temperatures through technical refinements to the experimental setup.
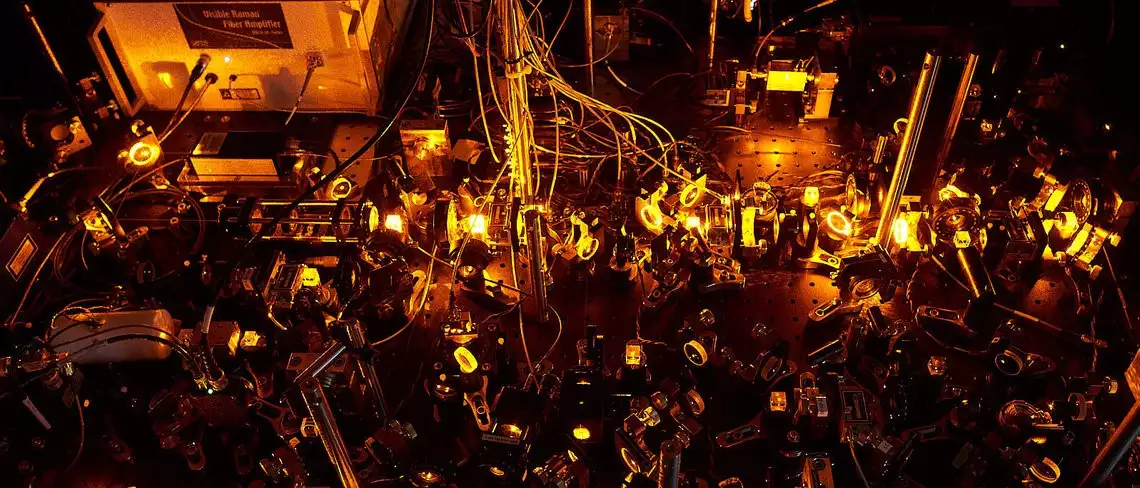
The findings may have significant ramifications for the study of quantum phenomena and quantum matter. According to researchers in the field of quantum optics - the new cooling technique is so simple that it can also be integrated into most experimental setups with ultracold polar molecules, so the method should soon find widespread application - and contribute to quite a few new findings. And it is claimed that the use of microwaves in conjunction with cooling "opens up a range of new inquiries into anomalous states of matter such as superfluids and supersolids. Additionally, it might be helpful for quantum technology. For instance, in quantum computers, where extremely cold molecules would be able to store data. For scientists studying ultracold polar compounds, these are genuinely exciting times - according to researchers in the field of quantum optics.