Cosmic Microwave Background Radiation:
Its Anisotropic Intentsity Across The Universe
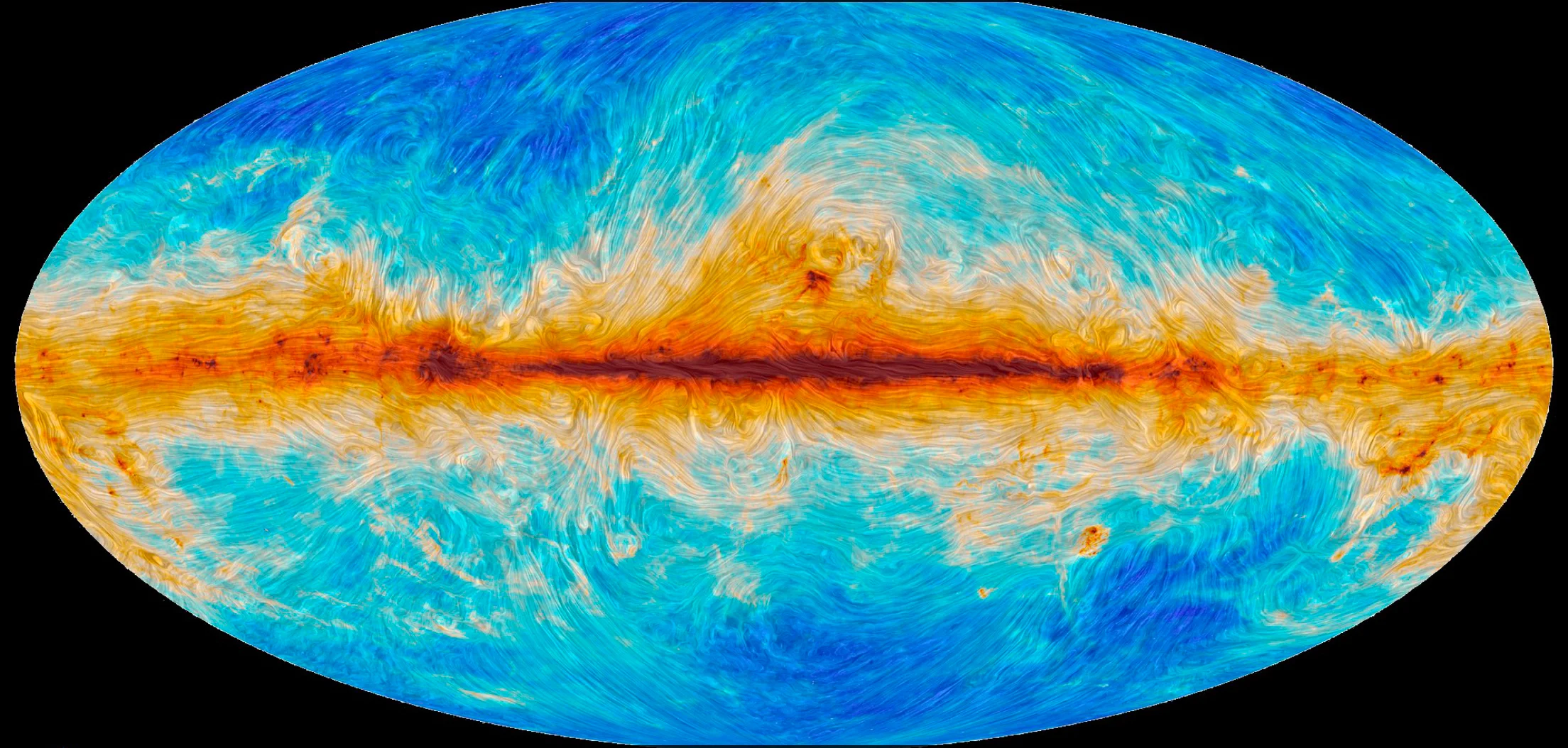
Cosmic microwave background radiation (CMBR) is a revealing informative residual from the very earliest time of the universe , almost from the birthing time of the universe. It was first detected in 1964. Its timeless existence is an irrefutable evidence that the universe originated from an extremely hot compressed point-sized origin or beginning. At the outset, Universe must have consisted of extremely hot radiation of plasma, elementary particles, photons (electromagnetic radiation) so as to have produced the CMBR which has almost uniform temperature and wavelengths in the radio region of the electromagnetic spectrum.
Currently the temperature of the CMBR is measured to 2.73°K. Research has found out that from the Universe'age of between 8 to 14 billion years, during all this epoch, Universe must has been expanding along with the CMBR cooling down. Its temperature today (2.73°K) indicates that most of the CMBR has changed to the radio energy in the microwave band. It is similar to microwaves bandwidth that scientists use for connection between the communication satellites and receiving stations on Earth.
But there are some key differences between the microwave bandwidth that scientists use and the CMBR.
→ CMBR is emanating from across the sky in all directions where as the band of microwaves that scientists use is transimitted from only one location in the sky.
→ CMBR consists of a wide range of microwave frequencies instead of being focused at one specific frequency for a radio transmitter to use.
→ CMBR consists of a wide range of microwave frequencies instead of being focused at one specific frequency for a radio transmitter to use.
Scientists needed to measure the CMBR over a wide spectrum of frequencies covering most of the sky to obtain precise information about the early universe. Though galaxies, stars and planets have started to form later on in the Universe, the CMBR has remained unchanged mostly, except that it has cooled down in course of time. So this CMBR is the most original residual from the early universe. So it has a great significance in understanding the origin and evolution of the universe from the outset.
The Radiation Spectrum Of The CMBR
The CMBR spectrum is expected to be in the range of thermal radiation which matches with the thermal spectrum from the Sun. BigBang theory correctly predicted the CMBR specturum. The themral spectrum has a specific configuration and its peak corresponds to the temperature of the body emitting the radiation. The CMBR at a temperture of 2.73°K peaks at 2-mm wavelength where as the Sun's thermal spectrum (at a temperatur of 6,000°K) peaks at a wavelength in the visible range. Experiments done over a several years with the ground-based and the baloon-based instruments lined up a rough sketch of the spectrum which supported the BigBang theory. However Scientists decided in the mid-1970's that to determine the CMBR spectrum decisively , the measurements needed to be carried out above the Earth atmosphere in the space , removing the obscuring casused by the atomsphere.NASA launched the Cosmic Background Explorer (COBE) to carry out these experiments in the outer space to trace out the thermal spectrum of the CMBR. The COBE provided the most accurate measurement of the spectrum in the first instance itself which matched the spectrum predicted by the BigBang. So this result led to the substantiation of the BigBang theory.
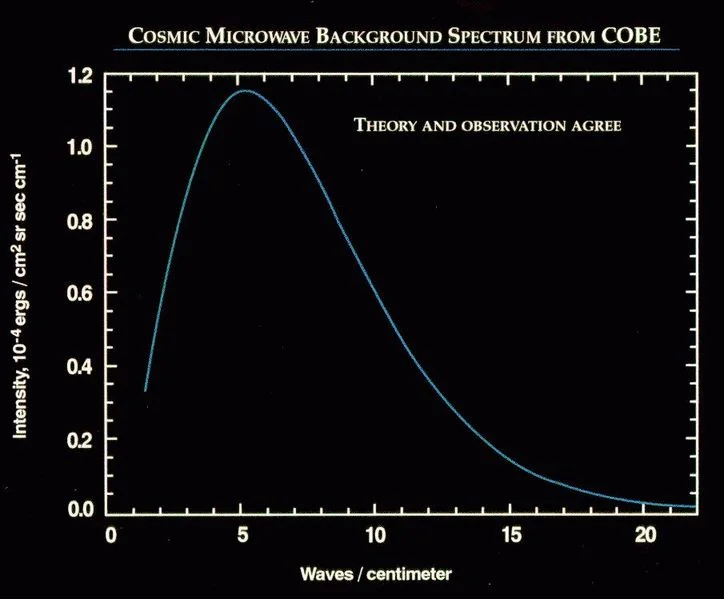
FIGURE-1: The thermal spectrum of CMBR which agrees with the prediction made by the BigBang.
This image shows the measurements of the spectral energy distribution of the CMBR. The measurements are perfectly described as the radiation from a body with a temperature of 2.728 K. The drawn curve corresponds to Planck's radiation law for this temperature. The measurement points follow the radiation law so well that they completely coincide with the drawn curve.
Image credit: NASA
This image shows the measurements of the spectral energy distribution of the CMBR. The measurements are perfectly described as the radiation from a body with a temperature of 2.728 K. The drawn curve corresponds to Planck's radiation law for this temperature. The measurement points follow the radiation law so well that they completely coincide with the drawn curve.
Image credit: NASA
The above image which shows the thermal spectum of the CMBR matches with the thermal spectrum of the blackbody radiation that Max Planck did research on and which led to the formation of the Quantum Mechanics. So this proves an interesting connection between the smallest and the largest realms of the matter. The reason for the specific shape of the thermal spectrum of the CMBR is that the entire Universe was at a uniform temperature for a considerable duration of its early history. The entire space was cluttered with plasma and thermal radiation which is the basis for the modern cosmology.
With one shot, the COBE satellite has settled the differing arguments about the thermal spectrum of the CMBR. But this is not an easy attempt. This required the transportation of the instrument in the satellite to above the Earth's atmosphere and the instrument temperature was made to reduce down to 1.5°K to prevent the instrument itself from emitting the radiation. Scientists took advantage of and made a good use of the experience and the knowledge gained in the operation of the ground-based and baloon-based instruments to design, operate and calibrate the COBE instrument.
The cosmological models on the basis of the BigBang theory predict that the temperature of the Universe goes down in course of time as the Universe goes on to expand. Observations made using the Keck telescope have supported the argument that the Universe'temperature has reduced over a period of cosmological time scale. By observing the light from a bright, distant, interstellar source, physists successfully measured the temperature of the corbon atoms in the interstellar clouds between the Earth and the source. They also guaged the shift in the spectral lines of the cloud . They found the temperature of the cloud to be 7.6°K in contrast to that it should have matched with the CMBR . But this is the exact temperature predicted at that redshift for the CMBR.
Fluctuations In The Intensity Of The CMBR
The intensity of the CMBR varies from location to location in the sky. These variaton measurements are called "anisotropic measurements". They point at the tiny changes in the uniform distribution of matter or radiation in the early Universe. These fluctuations are reckoned to be the seeds of the complex structures in the Universe that we observe today.The central argument is that the matter that we see today in the Universe such as galaxies, stars and planets must have formed due to the tiny fluctuations in the density of the radiation at the birth of the Universe. Gravity in those denser regions drew in matter together and the matter compressed enough over the time. As more and more matter accumulated together, the gravitional force in the matter increased significantly to cause the formation of galaxies and stars. These objects withstood any furhter collapse due to their internal motions or rotations.
This idea of tiny fluctuations in the density of radiation in the early stage of the Universe is called the "gravitational instability" which explains the presence of most of the astronomical objects in the sky today. Due to the expansion rate of of the Universe being very high, the formation of instabilites is greatly detained. So the seeds of the complex structures must have already been present at the last time that the CMBR acted on or interacted with matter which was probably 1,50,000 years after the BigBang. Even today, scientists are able to detect these tiny fluctuations through CMBR anisotropy measurements as tiny variations in the radiation intensity across the sky.
Large-Scale Anisotropy Measurements
Researchers were not able to meausre any anisotropy due to the lack of the high precision receiver sensitivity until 1992. After that for the first time, the COBE scientific team anounced that it had discovered the long-sought extrusions in the CMBR and it had released the COBE map of the intensity of the microwave radiation coming from various directions in the sky. The map incorporates some instrumental noise, but its clumps and extrusions indicate the probable causse of the beginnings of complex structures in the Universe.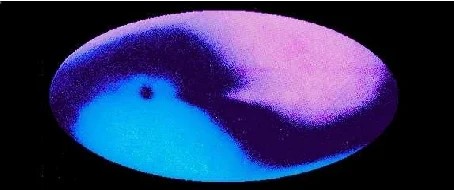
The CMB anistropies along with dipole and the Galaxy emission.
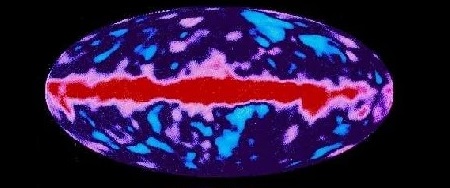
The dipole removed and CMB and Galaxy shown.
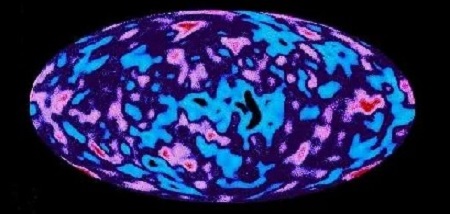
Only the CMB fluctuations shown.
Image credit: NASA
The experience gained in the earlier experiments on anisotropy from the ground, balloons and aircrafts helped scientists to discover the CMBR anisotropy with the COBE instrument. Along with this experience, the technology and physists' apprehension of how to evade the radiation contamination from the many bright local sources also evolved. Balloons, rockets and aircrafts provide the scientists with important opportunities for the detection of the CMBR radiation spectrum. Recently a balloon experiment detected the same CMBR pattern that was provided by the COBE by using a frequency above that of the COBE's receivers. This balloon experiment reconfirms the result provided by the satellite. These balloon experiments which are usually of low-cost helped scientists to run these results to smaller angular degrees.
The large-scale anisotropy enabled the CMBR research field to become firmly settled (established). The anisotropy detected by the COBE satellite is closely at the level required for the origin of complex structures that the theories predicted. Besides, COBE satellite measurements proved that the strength of the anisotropy varies with the angular size of the extrusions and it is consistent with the prediction made by the BigBang theory. COBE result even suggests an epoch of inflation in the early Universe though the corresponding CMBR fluctuations are not well detected yet. Since the announcement of the COBE result, cosmological theories have gone through an important transformation and they consider the COBE anisotropy signal as a reference point for futher developments.
Medium-Scale Anisotropy Measurements
Though the large-scale anisotropy detection helped in understanding the radiation density in the early Universe, it was carried out at angular scales of more than 10 degrees on the sky. But the formation of galaxies and clusters of galaxies happens at scales that are much smaller than 10 degrees. Instruments sensitive enough to detect the anisotropy reliably and give a mapping of CMBR anistotropy in a detailed way are yet to be developed. The smaller scales that these instruments have to adapt to are analogous to the physical scaling of superclusters of galaxies.Medium-scale anisotropy measurements which will be carried out at 0.5 to 10 degrees also enquire about the essential details of the decoupling process. Computer simulations show that at the time that radiation was combining together into atoms to form matter and interacted with CMBR lastly, there should have been acoustic oscillations in the over-compressed extrusions that should have given away powerful "fingerprints" in the CMBR anisotropy.
The theoretical prediction of extrusions in the CMBR medium-scale anisotropy gives us an interesting and unanticipated opportunity. If the examination of CMBR anisotropy versus extrusion size can be accomplished correctly , physicists may be able to evaluate three main cosmological parameters :
Ω - the ratio of the mean mass density to that required to have a closed Universe and stop its expansion finally
ΩB - the contribution to Ω from ordinary (baryonic) matter.
The expansion rate of the Universe (the Hubble constant Ho)
In addition, the data may disclose something very surprising, a hint at the unknown processes in the early Universe. Anyway, the medium-scale anisotropy measurements are going to give us detailed information about the processes and the dynamics that existed in the early Universe when it was only 1,50,000 years old. Medium-scale anisotropy measurements at angular scales around 1 degree (the low-end of the medium scale) have recently gained a lot of focus. But this scale equates with only about 300 million light years which is smaller than the region in the Uniserse around us that has been explored extensively in the redshift surveys of thousands of galaxies. Experiments on this samller scale have never been carried out before and hence are relatively new in comparision to the study of the CMBR spectrum and the large-scale anisotropy measurements. Physicists are just now beginning to understand the technology and methodology regarding how to overcome the new experimental challenges.
Physicists are making attempts regarding how to deal with the possible disturbance from the frail radio sources and the pervasive emission from our own galaxy. They are using better sensitivity tools and a wider range of frequencies to line up the separation. When the instruments can give the detailed mapping of large regions of the sky at angular scales around 1 degree, it is possible to get a good degree of understanding of the complex structures formation processes in the early Universe. There is much difficulty with the current experiments to accomplish this. In the case that ground-based instruments are used, they must deal with the fluctuations from the atmospheric emission which are thousands of times stronger than the expected CMBR anisotropy singnal. So it is best to carry out these experiments at cold dry atmospheric places such as the south pole, northern canada and mountain tops. By using the balloon-based experiments, it is possible to reduce the atmospheric fluctuations but they can afford only relatively short times for observations. Physicists are thinking up long-duration balloon flights of many days to cope with this problem and they also get a good opportunity to extend the COBE results at relatively low costs. But currently long-duration balloon flights are very scarce. Two other problems that physicists must deal with when they use the ground-based and balloon-based instruments are the limited sky coverage and the Earth's radiation environment.
Research is being done now to build a satellite that can take advantage of the space environment. The initial efforts indicate that a mid-sized explorer satellite orbiting around the Earth from a sufficient distance is a plausible solution. Using the satellite experiment, we can get the layout of the CMBR sky pattern with sufficient details and sensitivity which helps us understand how the development of the structure happend in the early Universe.