In the conventional view, "stuff" is made of atoms. The atoms are made of electrons, protons and neutrons. High Energy Physics deals with the question of what the electrons, protons and neutrons are made of. It is called "high energy" because experimentally one needs very high energy probes to try to take these "elementary particles" apart. By our current understanding, these elementary particles are excitations of the quantum fields that also govern their interactions.
By probing into the most basic componets of matter and radiation , Particle Physics (or the High Energy Physics) has discovered that these electrons , protons and neutrons are constituted of other "further more elementary" particles which are categorized into quarks, leptons, and bosons.
It involves deploying and calibrating particle accelerators , colliders and detetectors at various places in the world to proble into the deepest realms of matter. By exploring the most basic nature of space and time itself, it is increasing our knowledge of the universe enabling us to reach the farther most reaches of the cosmos thus revealing the purview of our existence here on earth.
It has uplifted the life of the humanity since the research work done in this field directly and indirectly influences various other braches of sciences like Quantum Physics, Nuclear Physics, AstroPhysics, Astronomy, Photonics and various other streams of engineering sciences and in turn, these sciences help the humanity in calibraing excellent useful innovations , thus improving the life on earth to thrive in various fields.
Research work in High Energy Physics involves the combined efforts of some of the largest scientific collaborations in the world, using some of the most sensitive detectors in the world, at some of the largest scientific machines in the world.
The Standard Model of the Particle Physics consists of six quarks, six leptons, four gauge bosons,
and one scalar boson (the Higgs boson), which interact through three interactions (strong force, weak force, and electromagnetism).
Contemporary Particle Physics also aims to explain the origin of mass and to converge all the various theories of the fundamental forces including gravity into a single unified framework which is rather called as "a theory of everthing".
High Energy Phsysics has found that the "normal" baryonic matter makes up only 4% of the universe's total energy and consequently the study of dark matter and dark energy has begun to take a great importance in the recent times.
FEATURED LINKS :
➤ Building Blocks Of The Universe
what is the universe made of? The short answer is that the universe is built of four substances: radiation, baryonic matter, dark matter and dark energy.
What are these substances?
1. Radiation: This consists of photons and neutrinos and makes up 10-3% of the total energy.
2. Baryonic matter: This is the ordinary, familiar material including all of the chemical elements and compounds that we find in planets, stars, gas clouds and plasmas. Baryonic matter only comprises about 4% of the universe's total entire energy.
➤ Black Holes And Curved Spacetime
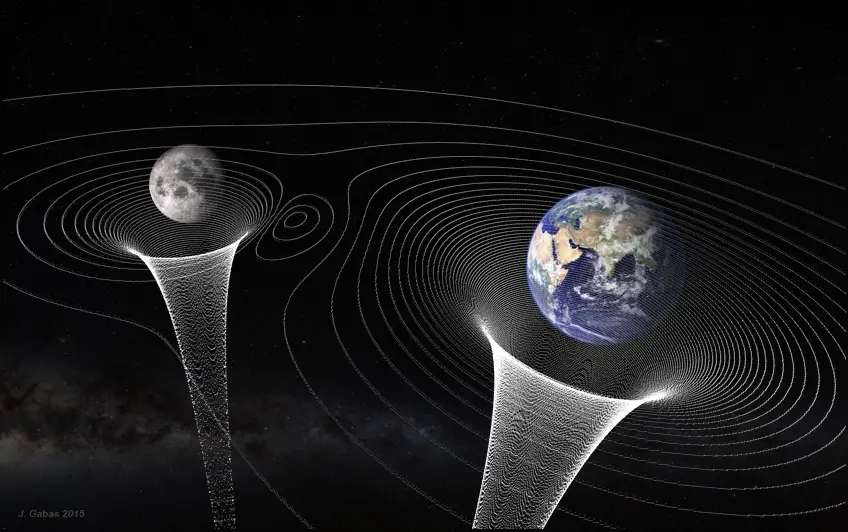
Most stars end their lives as white dwarfs or neutron stars. When a very massive star collapses at the end of its life, however, not even the mutual repulsion between densely packed neutrons can support the core against its own weight. If the remaining mass of the star’s core is more than about three times that of the Sun (\(M_{\text{Sun}}\)), our theories predict that no known force can stop it from collapsing forever! Gravity simply overwhelms all other forces and crushes the core until it occupies an infinitely small volume. A star in which this occurs may become one of the strangest objects ever predicted by theory—a black hole.
➤ Electron - Higgs Field Interaction
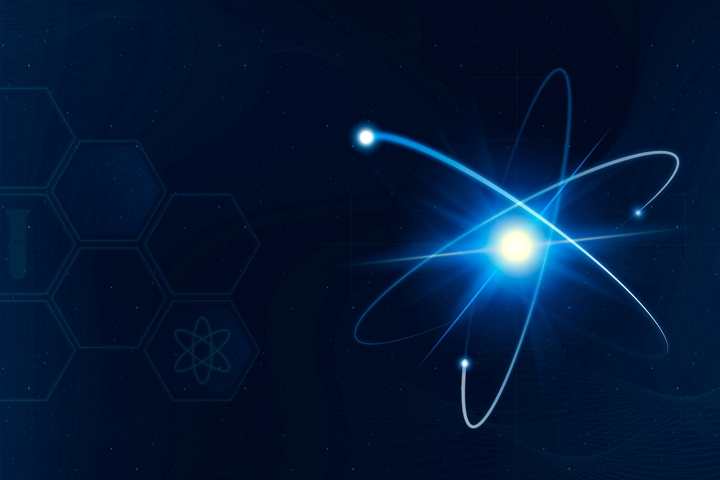
The Higgs boson (a particle of the Higgs field) is a particle associated with the electroweak-symmetry breaking mechanism which is an inmportant aspect of quantum physics and is a vital component of the Standard Model of particle physics. It is believed that all fundamental particles in the known Universe that have mass — for example electrons, or the quarks that live inside protons and neutrons — acquire this mass as a result of interacting with an omnipresent field through Higgs bosons. Massless particles, such as photons, pass through the field without interacting with it.
Everything that we can touch and see and feel has substance. All this substance that makes up our Universe is courtesy of the familiar atom, which gives us all the matter around us. An atom is comprised of lightweight electrons orbiting and bound to a bulky nucleus of protons and neutrons, which themselves are made of quarks.
➤ Matter - Antimatter Symmetry
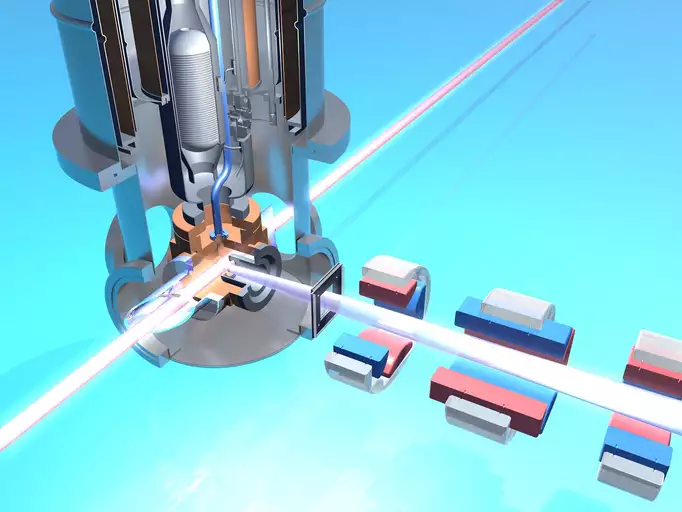
According to the Standard Model of elementary particle physics, to each particle exists an antiparticle that is supposed to behave exactly the same way. Thus anti-particles which make up the anti-matter would observe the same laws of physics in general, as the matter particles do. This phenomena is, however, difficult to prove, since it is almost impossible to perform measurements on antimatter: whenever an antiparticle meets is matter-counterpart, both particles annihilate, accompanied by the creation of energy.
A recent research in Quantum Optics has found a way to overcome this hurdle: an experiment was carried out by trapping an antiproton inside a helium atom. As due to a new cooling technique the helium atoms are almost at rest, high precision spectroscopy measurements are made possible. For the mass of the antiproton relative to the electron, the outcome of the research has achieved the unprecedented accuracy of 800 parts per trillion.